Study Directly Ties BP Oil Rig Explosion to Dramatic Rise in Dolphin Deaths.

According to the study published by the online scientific journal Plos One, the mortality rate of bottlenose dolphins in the Gulf began increasing around February 2010 and continued into last year, “overlapping in time and space with … the Deepwater Horizon oil spill.”
Dolphins found dead along the Gulf coast were tested for evidence of biotoxins, morbillivirus, and brucellosis and results were compared to dolphins from outside the region. Scientists found that “an unusually high number of dead Gulf dolphins had what are normally rare lesions on their lungs and hormone-producing adrenal glands.”
In fact, one out of every three dead Gulf dolphins had these lesions on their adrenal glands, causing a serious condition known as adrenal insufficiency, which leaves animals “less able to cope with additional stressors in their everyday lives.” This inability to cope with added stressors makes the affected animals more likely to die.
“This is the latest in a series of peer-reviewed scientific studies, conducted over the five years since the spill, looking at possible reasons for the historically high number of dolphin deaths that have occurred within the footprint of the Deepwater Horizon spill,” said NOAA’s Dr. Terri Rowles, one of the study’s authors.
“These studies have increasingly pointed to the presence of petroleum hydrocarbons as being the most significant cause of the illnesses and deaths plaguing the Gulf’s dolphin population,” said Dr. Rowles.
In the five years since the disaster, BP has not only tried to avoid taking responsibility for the damages its rig explosion caused, it has tried to downplay the effects the spill actually had on the environment.
Last month, the oil giant sent out a report basically claiming “all is well” in the Gulf and things are returning to normal. This study, along with numerous others, proves that untrue. The Gulf is still very much affected by the spill — humans and animals alike.
“While dolphins and our ecosystem continue to die a BP death, most corporate media refuses to report the story,” said Ring of Fire host Mike Papantonio. “That’s what huge advertising dollars will do for psychopaths like British Petroleum. They have all the money, and we are left with all the suffering.”
Less Than 50 Maui's Dolphins Left.
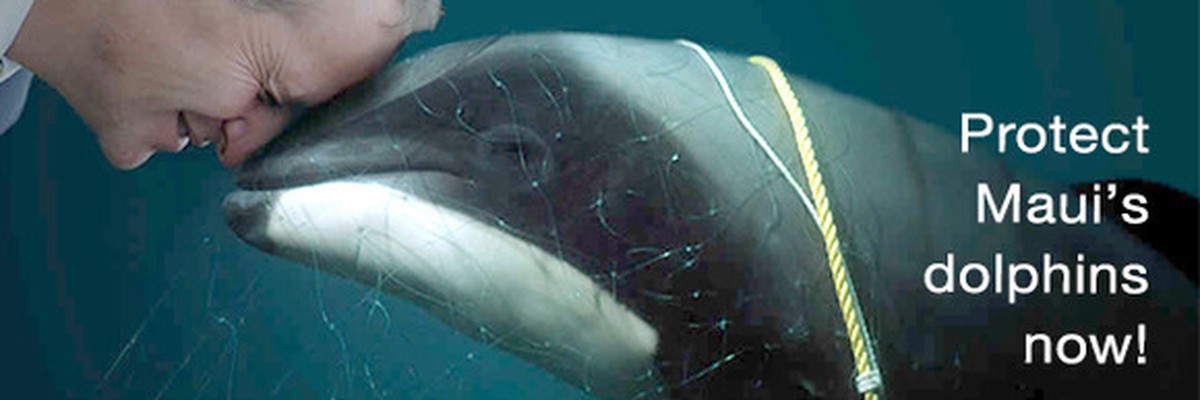
Maui's dolphin numbers have dropped below 50 and now sit at an all time low of between 43 and 47, a New Zealand researcher presenting new figures to an overseas summit says.
University of Otago Professor Liz Slooten, who is taking part in discussions with 200 leading cetacean scientists at the International Whaling Commission's Scientific Committee in San Diego, also estimated there now just 10 to 12 adult female Maui's dolphins.
She warned that unless the level of fisheries protection was increased significantly, the critically-endangered dolphins could become extinct in just 15 years.
The subspecies of Hector's dolphins, found in shallow coastal waters up to depths of 100 metres off the North Island's west coast, have become a symbol for environmentalists challenging gill netting and trawling by commercial fishers, and Government oil and gas exploration block offers in habitat areas.
Earlier this year, Auckland councillors voted to oppose oil exploration in a sanctuary home to the dolphin, but stopped short of following Christchurch City Council and opposing any exploration, while a survey suggested New Zealanders would be happy to pay for greater protection for the dolphins.
Maui's dolphin numbers - which environmentalists have generally put at 55 and the Department of Conservation has estimated at between 48 and 69 - had dropped 97% as a result of fishing since the 1970s, NABU International Nature Conservation Foundation said in a statement.
The group stated the numbers had dropped from 111 in 2004 to 59 in 2010/11, and claimed the absence of man-made deaths, such as dying in fishing nets, would set the dolphins back on the road to recovery and allow numbers to grow to 500 individuals in 87 years.
photo via HectorsDolphins.com
Because Maui's dolphins could only cope with one human-induced death every 10 to 20 years, immediate conservation measures are urgently required, NABU said.
There has been debate around figures surrounding the dolphin's population and its extinction deadline.
Photo by KASM
Last year, a Ministry for Primary Industries spokesperson told the New Zealand Herald that while there was no debate numbers were at a "very low level", the Government had not seen any analysis or evidence that supported research suggesting existing protection measures would lead to the Maui dolphin's functional extinction within the next two decades.
NABU International's head of endangered conservation species, Dr Barbara Maas, said the new figures were an "unmistakable wake-up call", arguing New Zealand had to stop placing the interests of the fishing industry above biodiversity conservation.
At present, the Government has in place a range of set net, trawling and drift net restrictions throughout the dolphins' habitat, while there are also restrictions on seabed mining and acoustic seismic survey work within the boundaries of the West Coast North Island Marine Mammal Sanctuary, which was extended in 2013 to include more of the Maui dolphin's range in the Taranaki area.
The Government was also reviewing a threat management plan already in place for the dolphins, with the programme to be informed by a marine research and advisory group of scientific and stakeholder experts.
Take action here to save the last 50. Make a new thunderclap and support HectorsMauisSOS and NABU International, who protects endangered species and their habitats.
World’s Rarest Porpoise Is Dying to Feed a Black Market in Fish Bladders. A new Greenpeace investigation links Mexico, the United States, and Hong Kong in the illegal wildlife trade killing off the vaquita. 
A wildlife black market stretching from Mexico’s Sea of Cortez to the streets of Hong Kong will drive the world’s most endangered porpoise to extinction unless it is shut down soon, according to a new report.
In the investigation, published on Wednesday, Greenpeace investigators said that vaquita porpoises are dying after being caught and drowned in illegal gill nets in the northern Gulf of California, the marine mammal’s only habitat.
The vaquita population has fallen from 200 porpoises in 2012 to 97 today, said Miguel Soto Treviño, the communications coordinator for Greenpeace Mexico.
The Mexican government banned fishing in vaquita habitat in April, but illegal fishing has surged in the region with rising demand in China for the bladder of a fish called the totoaba.
“In 2013 people from China appeared with thousands of dollars in their bags, in cash,” according to fishers in the region, said Treviño, and they are “paying Mexican fishermen $3,000 to $9,000 per kilogram of totoaba bladders.”
The totoaba itself is listed as an endangered species in Mexico and the U.S. because of overfishing.
Greenpeace’s investigators found that a single totoaba swim bladder can sell for $5,000 to more than $15,000 at shops in Hong Kong. A bladder weighing 400 grams can fetch $65,000, said Treviño.
Mexican couriers employed by Chinese smugglers transport the bladders into the United States, said Treviño, and then fly them from San Francisco or Los Angeles to dealers in Hong Kong. Customs officials there have either not discovered the illegal products or are overlooking the trade.
This makes the governments of all three countries responsible for the survival of the vaquita, he said. “Mexico made the first step with the fishing ban,” said Treviño. “Now we want cooperation between Hong Kong and United States authorities to stop this situation.”Some people in China believe that the bladder, cooked in a soup, improves fertility and virility, said Treviño.
Businessmen are using these bladders as special gifts to authorities, to high-profile politicians, to strengthen their relationships—as a way to show power and wealth,” he added, while other “want to keep that bladder because they know the price of the bladders will increase if the species is almost extinct.”
Greenpeace released the report simultaneously in the United States, Mexico, and Hong Kong on Wednesday, hoping to increase public awareness of the vaquita’s plight, as well as pressure governments to stop the bladder black market that is indirectly pushing the porpoise to extinction.
“I think that U.S. citizens can demand to their own government, ‘Stop contributing by omission to the extinction of a cetacean of Mexico,'” Treviño said.
So, Why Should You Care? Scientists estimate that 25 percent of the world's mammals are at risk of extinction. Saving them will mean restoring healthy environments that benefit animals and humans alike. One indicator of ecosystem health is biodiversity: the scope of variety in the plants and animals that live in a particular habitat. Losing a top-of-the-food-chain predator like the vaquita porpoise could have serious impacts on the ecological balance lower down the food chain, possibly affecting fish stocks that humans depend on for food.
Last Hope for the Vaquita Late last year the International Committee for the Recovery of the Vaquita (CIRVA) estimated that fewer than 100 vaquitas were left in the Upper Gulf of California, the only place on Earth where they are found. They also stated that if the different fisheries in region did not stop accidentally catching and killing these animals (which is called bycatch), the critically endangered vaquita had only four more years before becoming extinct. Fortunately, new measures decreed by the Mexican government are giving the vaquita its last hope to survive.
The vaquita’s range is limited to just a small section of the Gulf of California 
In April, Mexico decreed a two-year ban on fishing activities throughout the vaquita’s range in the Upper Gulf. The only fishing that will be allowed in the area is for curvina. Boats trying to catch curvina use a type of fishing gear that does not capture vaquitas. Such a wide-reaching ban marks a serious commitment from the Mexican government to try to save this unique animal before it is lost forever.
Inspectors from the Environmental Enforcement Agency, Fishery authorities and the Navy will all be in charge of enforcing the ban. The President of Mexico delivered a new fleet of fast patrol boats to the Navy to make sure they are able to track down and stop any illegal fishing boat, and they will also be using drones for surveillance of the whole area. In the past, illegal fishing has been just as much a problem for the vaquita as legal fishing, so having these new resources to police the waters will hopefully make a big difference.
Measures like this fishing ban can have economic impacts on those who rely on fishing to make their living. So to encourage fishermen to comply with the ban, the Mexican government will compensate them for their loss of income. It is estimated that as a whole, fishermen will be receiving around $50 million dollars each year. This way, the vaquita can be given a fighting chance, but the economic impact on fishermen can be decreased.
The ban will be in place for two years. This is to give shrimpers enough time to change to a fishing gear that does not affect vaquitas. That change is a mandatory part of the new measures, given that the shrimp fishery is the legal fishery that captures the most vaquitas.
All in all, these measures are good news. In fact, they are the last hope for the vaquita to survive. If they don’t work or aren’t implemented correctly, and the vaquita numbers continue to decrease, there will be nothing left that we can do.
Illegal fishing continues to be the largest threat. It has been estimated that 40% to 50% of the fleet fishing for shrimp, shark, mackerel, ray and other fin fish in the Upper Gulf consists of illegal fishermen that have no fishing permit. These fishers are not part of the compensation plan, and surely they could care less about the ban since they already fish illegally. Organized crime, which is already prevalent in the region’s illegal totoaba fishery, adds another layer of complication to the problem.
For these latest measures to truly help the vaquita, the key will be to fully enforce the ban during the next two years. We hope that the additional resources the Mexican government has provided will make enforcement easier, more effective, and more widespread. In the meantime, we will continue to advocate for the ban to be extended beyond just two years, and to expand the vaquita refuge area. It will also be essential to reduce the demand for the products gained through the illegal fishing, like the totoaba demand in China. Last year, Mexico and the U.S. presented a joint statement at the 65th meeting of the International Whaling Commission calling on all nations to join in the effort to stop the illegal trade and save the vaquita. Only by reducing demand can we hope to also reduce this harmful practice. This part of this post Last Hope for the Vaquita appeared first on Defenders of Wildlife Blog.
The California coast is drenched in oil from a shoddy pipeline rupture, while Plains All American CEO Greg Armstrong raked in over $5 million in compensation last year, and is guaranteed $29 - $87 million in golden parachute cash! Enough is enough, dolphins are dying. Sign now calling on California’s Attorney General to file charges: SIGN THE PETITION |
While our rocky shores are awash in oil and dead fish, Plains All American CEO Greg Armstrong raked in over $5 million in compensation last year, and is guaranteed $29 - $87 million in golden parachute cash! These guys broke the law to make a quick buck. But if we hold them accountable, we can prevent another catastrophe by putting oil company executives everywhere on notice that they can’t get away with these kinds of shady games on our watch.
Let's tell California Attorney General Kamala Harris and local District Attorney Joyce Dudly to file civil and criminal charges against Plains All American and its shady CEOs! Sign now, and spread the word:
https://secure.avaaz.org/en/santa_barbara_oil_spill_loc/?bIQwIeb&v=59386
The pipeline that burst lacked basic safety features, like an automatic shut-off valve. And Plains All American is one of the most reckless companies in the US, with over 175 documented violations in the past decade. They’ve been warned time and again, but did nothing.
I live in San Diego, just 200 miles south of this devastating oil spill. My best days are the days I surf with seals and dolphins. Floating in the waves as they frolic with joy and abandon makes the whole world make sense.
These precious creatures are now drowning in oil because of the profiteering short cuts of the Plains All American, but we can hold the culprits accountable. Click now to tell the DA and AG to bring the maximum possible charges:
https://secure.avaaz.org/en/santa_barbara_oil_spill_loc/?bIQwIeb&v=59386
50 years ago a similar oil spill devastated Santa Barbara’s coastal sanctuary, sparking the modern environmental movement around the world. Together people everywhere rose-up then in fury and hope, writing new laws to protect our planet and our children’s future. Let us allow this tragedy to renew our determination, so together we can rise again.
More information:
Santa Barbara oil spill: Authorities, environmentalists step up response (CNN)
http://www.cnn.com/2015/05/22/us/california-oil-spill
Santa Barbara oil pipeline leak rekindles memories of 1969 disaster (LA Times)
http://www.latimes.com/local/california/la-me-santa-barbara-spill-20150521-story.html#page=1
Huge Oversight Gap on Refugio Pipeline (Santa Barbara Independent)
http://www.independent.com/news/2015/may/21/whos-watching-man-whos-watching-pipeline
SEC Form 10-K Annual Report (SEC)
http://www.sec.gov/Archives/edgar/data/1070423/000110465915013616/a14-25277_110k.htm#Item11_Executiv...
Executive Profile (Boardroom Insiders)
http://people.equilar.com/bio/greg-armstrong-plains-all/salary/25718#.VWYDse0azCQ
Read the full NOAA study here:
Adrenal Gland and Lung Lesions in Gulf of Mexico Common Bottlenose Dolphins (Tursiops truncatus) Found Dead following the Deepwater Horizon Oil Spill
Abstract
A northern Gulf of Mexico (GoM) cetacean unusual mortality event (UME) involving primarily bottlenose dolphins (Tursiops truncatus) in Louisiana, Mississippi, and Alabama began in February 2010 and continued into 2014. Overlapping in time and space with this UME was theDeepwater Horizon (DWH) oil spill, which was proposed as a contributing cause of adrenal disease, lung disease, and poor health in live dolphins examined during 2011 in Barataria Bay, Louisiana. To assess potential contributing factors and causes of deaths for stranded UME dolphins from June 2010 through December 2012, lung and adrenal gland tissues were histologically evaluated from 46 fresh dead non-perinatal carcasses that stranded in Louisiana (including 22 from Barataria Bay), Mississippi, and Alabama. UME dolphins were tested for evidence of biotoxicosis, morbillivirus infection, and brucellosis. Results were compared to up to 106 fresh dead stranded dolphins from outside the UME area or prior to the DWH spill. UME dolphins were more likely to have primary bacterial pneumonia (22% compared to 2% in non-UME dolphins, P = .003) and thin adrenal cortices (33% compared to 7% in non-UME dolphins,P = .003). In 70% of UME dolphins with primary bacterial pneumonia, the condition either caused or contributed significantly to death. Brucellosis and morbillivirus infections were detected in 7% and 11% of UME dolphins, respectively, and biotoxin levels were low or below the detection limit, indicating that these were not primary causes of the current UME. The rare, life-threatening, and chronic adrenal gland and lung diseases identified in stranded UME dolphins are consistent with exposure to petroleum compounds as seen in other mammals. Exposure of dolphins to elevated petroleum compounds present in coastal GoM waters during and after the DWH oil spill is proposed as a cause of adrenal and lung disease and as a contributor to increased dolphin deaths.
Figures
Citation: Venn-Watson S, Colegrove KM, Litz J, Kinsel M, Terio K, Saliki J, et al. (2015) Adrenal Gland and Lung Lesions in Gulf of Mexico Common Bottlenose Dolphins (Tursiops truncatus) Found Dead following the Deepwater Horizon Oil Spill. PLoS ONE 10(5): e0126538. doi:10.1371/journal.pone.0126538
Academic Editor: Cheryl S. Rosenfeld, University of Missouri, UNITED STATES
Received: December 22, 2014; Accepted: March 30, 2015; Published: May 20, 2015
This is an open access article, free of all copyright, and may be freely reproduced, distributed, transmitted, modified, built upon, or otherwise used by anyone for any lawful purpose. The work is made available under the Creative Commons CC0 public domain dedication
Data Availability: All relevant data are available within the paper and Supporting Information files.
Funding: This study was funded by the Deepwater Horizon National Resource Damage Assessment being conducted cooperatively among the National Oceanic and Atmospheric Administration (NOAA) (www.noaa.gov), other Federal and State Trustees, and BP; the Contingency Fund of the Working Group for Marine Mammal Unusual Mortality Events (WGMMUME)(http://www.nmfs.noaa.gov/pr/health/mmume/history.htm); National Fish and Wildlife Foundation (www.nfwf.org); and NOAA’s Marine Mammal Health and Stranding Response Network (http://www.nmfs.noaa.gov/pr/health/). NOAA and the WGMMUME aided in reviewing the study design and manuscript. BP funders had no role in study design, data collection and analysis, decision to publish, or preparation of the manuscript.
Competing interests: This work was part of the Deepwater Horizon National Resource Damage Assessment being conducted cooperatively among NOAA, other Federal and State Trustees, and BP. This does not alter the authors' adherence to PLOS ONE policies on sharing data and materials.
Introduction
A large, multi-year cetacean unusual mortality event (UME) has been ongoing in the northern Gulf of Mexico (GoM) since February 2010, continuing into 2014 [1]. This event has involved predominantly (87%) common bottlenose dolphins (Tursiops truncatus) (hereafter referred to as ‘dolphins’) stranded in Louisiana, Mississippi, and Alabama [2]. The UME coincided with theDeepwater Horizon (DWH) oil spill, the largest marine-based spill in U.S. history [3]. During and following the DWH oil spill, significantly elevated polycyclic aromatic hydrocarbon (PAH) levels attributed to this spill were detected in coastal GoM waters, including Louisiana, Mississippi, and Alabama [4]. These locations coincided with the states most impacted by the ongoing UME since the DWH oil spill [2]. Dolphin strandings, however, were elevated during March and April before the spill, necessitating an investigative approach including numerous potential causes [1,2,5]. Combined oil exposure, an unusually cold winter during 2011, and fresh water infusions have been proposed as potential causes contributing to this UME [6].
Barataria Bay, Louisiana was one of the heaviest oiled coastal areas from the DWH oil spill, including visualized oiling from the spill encompassing 40 km and 366,000 m2 of Barataria Bay’s shoreline lasting in decreasing amounts for at least 2 years [7–10]. The presence of increased coastal PAH levels associated with the DWH oil spill, especially near Grand Isle, Louisiana in Barataria Bay have been confirmed [4]. Further, within the time period of January 2010 to June 2013, the longest lasting cluster of dolphin strandings throughout the northern GoM was in Barataria Bay (August 2010 through 2011) [2]. During the DWH oil spill and response period, numerous dolphins, including dolphins in Barataria Bay, were observed swimming through visibly oiled waters and feeding in areas of surface, subsurface, and sediment oiling [11].
Due to the extensive oiling in Barataria Bay, health assessments were conducted on live dolphins in this area during the summer of 2011 [11]. Barataria Bay dolphins had a high prevalence of moderate to severe lung disease and blood value changes indicative of hypoadrenocorticism; specific blood changes included low serum cortisol, aldosterone, and glucose, and high neutrophil counts [11]. Nearly half (48%) of Barataria Bay dolphins were given a guarded to grave prognosis for long-term survival [11]. The DWH oil spill was proposed as a contributor to adrenal gland and lung disease in live Barataria Bay dolphins.
Previous to the ongoing event, there have been ten dolphin GoM UMEs since 1991, as well as one large die-off during 1990 that occurred before the UME declaration process [1, 12–15]. The majority (82%) of previous dolphin GoM events had brevetoxicosis or morbillivirus as confirmed or suspected causes [1]. While brevetoxicosis events do not leave a histologic signature in affected dolphins, brevetoxicosis-related events are often associated with known algal blooms and deaths that appear to be acute in otherwise healthy-looking dolphins [15]. In prior events classified as brevetoxicosis-related, 50% or more sampled dolphins were positive for brevetoxin with most at high concentrations [15]. Similarly, past UMEs that have been attributed to morbillivirus involved successful detection of morbillivirus in greater than 60% of dolphins tested. [13,16]. There is evidence that Brucella, which is commonly found in marine mammals worldwide, can cause disease in cetaceans, including bottlenose dolphins [17–21]. As such, there was a need to evaluate all of these potentially important diseases as playing contributing or leading roles in the ongoing UME.
To assess contributing factors and causes of deaths for stranded UME dolphins following the DWH oil spill, tissues were histologically evaluated from 46 carcasses that stranded in Louisiana, Mississippi, and Alabama, including 22 from Barataria Bay, from June 2010 through December 2012. Perinatal dolphins, stranded dolphins that were less than 115 cm in body length that likely died during late-term pregnancy or shortly after birth, were excluded from this study. On the basis of the live dolphin health assessment findings from Barataria Bay, this study included a focused evaluation of adrenal, lung, and liver lesions with the expectation that if stranded dolphins had been impacted by the DWH oil, they would have lesions consistent with the clinical evidence indicating lung disease and hypoadrenocorticism found in live dolphins. Other potential causes of and contributors to dolphin deaths were investigated, including the presence of histologic lesions and diagnostic test results consistent with brevetoxicosis, morbillivirus infections, and brucellosis. Results were compared to a reference group of fresh dead dolphins from North Carolina, South Carolina, Texas, and the Gulf coast of Florida that stranded prior to or remote from the UME and DWH oil spill timeframes and geographic location.
Materials and Methods
Tissues and data from bottlenose dolphins that stranded dead or stranded and died were used in this study in coordination with NOAA’s Marine Mammal Health and Stranding Response Program (MMHSRP, T. Rowles) and NOAA’s Southeastern United States Marine Mammal Stranding Network. Only samples from stranded dolphins were included in this study. No animal was killed for the purposes of this study.
UME dolphins
This study includes common bottlenose dolphins (Tursiops truncatus) with a total body length greater than or equal to 115 cm that stranded in Louisiana, Mississippi, or Alabama from June 2010 through December 2012. Dolphins with a total body length less than 115 cm were likely either near-term pregnancy losses or died soon after birth. Carcasses included in this study were either characterized as stranding fresh dead by the responding stranding network personnel or stranded alive then died, had archived tissues available for histologic evaluations, and had tissues not compromised by decomposition based on histologic evaluations by a pathologist. Fresh dead dolphins were the focus of this study to enable the most reliable histologic evaluations which could be compared to other diagnostic test results. Blood-based clinical pathology data were not available from dead, stranded dolphins as a direct comparison to the indices of hypoadrenocorticism detected in the previously published, live dolphin health assessment.
Reference dolphins
A total of 106 fresh dead reference dolphins, each with a total body length greater than or equal to 115 cm and an existing histopathology report, were compared to UME dolphins in this study [22]. Of these, 51 (48%) reference dolphins had archived tissues available for standardized assessment by a common pathologist (K. Colegrove) heretofore called the ‘standardly scored reference subset’. Reference dolphins for this study stranded in North Carolina and South Carolina from 1996 through 2012, or from Texas or the Gulf coast of Florida from 2002 through 2009 (Table 1). Histologic lesion comparisons from reference dolphins were made to the UME dolphins using both the full reference group and the standardly scored reference subset group. It is possible that some of the reference dolphins may have been part of historical UMEs, specifically brevetoxin UMEs in Florida. Potential brevetoxicosis reference dolphins were not excluded from this study to ensure there was no bias against this as a cause of the ongoing UME.
Table 1. Total number, stranding dates, and locations of 152 freshly dead stranded non-perinatal (greater than or equal to 115 cm in total body length) common bottlenose dolphins (Tursiops truncatus) from the ongoing northern Gulf of Mexico cetacean Unusual Mortality Event (UME) and reference groups.
doi:10.1371/journal.pone.0126538.t001
Sample collection and processing for histologic examination
Post mortem examinations of UME and reference dolphins were conducted by members of NOAA’s Southeastern United States Marine Mammal Stranding Network either in the field or at institutional facilities. Stranding demographic, temporal and spatial data were collected using a standardized Level-A data record (NOAA Form 89–864 (rev.2007) OMB No. 0648–0178) according to approved NOAA protocols at the time of data collection. Level A data used for this study were the most recently available when extracted from the NOAA’s MMHSRP database during September 2014.
Tissue samples from most major organs of stranded dolphins were collected in 10% neutral buffered formalin, many of which included adrenal glands, bladder, brain, eye, heart, kidney, liver, lung, lymph node(s), muscle, pancreas, reproductive organs, skin, small and large intestines, spinal cord, spleen, thymus, tongue, and trachea. Tissues were processed routinely, embedded in paraffin, sectioned at 5 μm, and stained with either hematoxylin and eosin (HE) (UME dolphins, South Carolina reference dolphins, and Gulf coast of Florida reference dolphins) or hematoxylin, phloxine, and saffron (HPS) (Texas reference dolphins). While this study focused on lung, adrenal gland, liver, lymph nodes and brain to test our hypothesis, full sets of tissues from the UME cases, including the above organs, were evaluated by a pathologist and used for the cause of death determination. Among the 10 UME dolphins with primary bacterial pneumonia, gram stains were completed on all dolphins, and Ziehl Nielson acid fast stains were completed on lung sections of four dolphins. Not all tissues for all dolphins were available for both histologic and other diagnostic tests (e.g. there may have been formalin-fixed lung for histologic evaluation, but no frozen lung for morbillivirus molecular diagnostics). As such, the total numbers of tissues varied throughout the study based upon their availability for each investigation.
Histologic grading
Targeted evaluation of lesions in select organs (adrenal gland, brain, lung, lymph node, spleen, and liver) from UME dolphins was performed by a board certified veterinary pathologist (K. Colegrove) through evaluation of HE or HPS stained slides. To standardize comparisons among tissues, the same evaluation was completed on the reference dolphins by the same pathologist (K. Colegrove) through evaluation of stained slides (n = 51, standardly scored reference set) or thorough interpretation of lesion descriptions with or without images from histopathologic reports (n = 55) written by other pathologists. To reduce bias, severity grades were carried out blindly without knowledge of the animal identification number.
Adrenal gland.
The adrenal gland was evaluated quantitatively by measurement of corticomedullary ratios (thickness of cortex divided by thickness of medulla). Corticomedullary ratios were determined similarly to previously published methods on mid-sagittal or mid-cross sections of adrenal glands from 36 UME and 44 reference dolphins using an Olympus BX-41 microscope and Olympus DP-BSW Version 03.02 measuring software [23]. Corticomedullary ratios in dolphins remain constant along the length of the adrenal gland, indicating that ratios calculated on mid- sagittal or mid-cross sections are adequately representative of the entire adrenal gland [24]. Three corticomedullary ratios were obtained from three different representative sites along the section and averaged. For most UME and reference dolphins, only one appropriate mid-sagittal or mid-cross section of either right or left adrenal gland was available for evaluation. Adrenal corticomedullary ratios from 44 reference dolphins were used to statistically define ‘thin’ and ‘thick’ adrenal gland cortices. Thin was defined as a corticomedullary ratio less than the 10thquantile among reference dolphins (0.447), and thick was a corticomedullary ratio greater than the reference’s 90th quantile (0.838). UME and reference dolphins were excluded from evaluation of corticomedullary ratios when mid-sagittal sections of adrenal glands were not present or sections had been cut in a way that precluded precise measuring of cortex or medulla thickness. Corticomedullary ratios were obtained from both the left and right adrenal glands of ten reference dolphins, and using a Fisher’s exact test, measurements were comparable between the left and right sides (P = 0.85). Presence or absence of vacuolation of corticocytes in the zona fasciculata was also evaluated.
Lung.
Lung inflammation severity was graded as mild, moderate, or severe based on extent and number of inflammatory cells. Mild lesions were those in which there were small and often multifocal accumulations of small numbers of inflammatory cells with minimal disruption of parenchyma. Moderate lesions were multifocal to more widespread, had moderate accumulations of inflammatory cells, and moderate disruption of parenchyma. Severe lesions had regional to diffuse accumulations of large numbers of inflammatory cells that often completely obscured or disrupted parenchyma. The distribution of the inflammation was defined as bronchopneumonia, bronchointerstitial pneumonia, or interstitial pneumonia. The types of inflammatory cells present within the inflammatory lesions were denoted as neutrophilic, eosinophilic, lymphoplasmacytic and granulomatous and were not mutually exclusive. Lesions were evaluated for the visual presence of bacterial and fungal organisms.
The cause of inflammation was defined as primary bacterial if bacterial organisms were visualized and associated with lesions and there was no concurrent evidence of lungworm, fungal or viral infection contributing to the inflammation. Inflammation was defined as primary lungworm if lesions were consistent with dolphin lungworm infection as previously described [25] and there was no concurrent or secondary bacterial, fungal, or viral infection. Inflammation was defined as primary fungal or viral-associated if the pathogen was visualized without other concurrent pathogens. Mixed infections were those in which there were multiple infectious agents (e.g. bacteria and lungworm) associated with the inflammatory lesions.
Pulmonary fibrosis was graded based on extent and frequency of lesions. Mild lesions were those in which lesions had a patchy distribution (less than 30% of the parenchyma was affected), and alveolar septae and/or perivascular connective tissue were mildly thickened by increased amounts of collagenous matrix. Moderate lesions were those which had a more widespread distribution (30–60% of parenchyma affected), alveolar septae and/or perivascular connective tissue moderately thickened by increased amounts of collagenous matrix, and/or thick bands of fibrous connective tissue with moderate disruption of pulmonary parenchyma. Severe lesions had large areas of parenchyma affected (greater than 60%), marked thickening of alveolar septae and/or perivascular connective tissue by increased amounts of collagenous matrix and large areas in which normal architecture was completely obliterated by fibrous tissue. Angiomatosis was defined as a proliferation of small thick-walled vessels, similar to lesions previously described at a prevalence of 46% in dolphins stranded in Texas from 1991 to 1996 [26]. Lungworm-associated pulmonary granulomas, when present, were defined as active or chronic similarly to what has been previously described in GoM dolphins [25].
Other histologic evaluations.
Degree of inflammation in the central nervous system was subjectively graded as mild, moderate, or severe based on extent and number of inflammatory cells and frequency of inflammatory foci in tissues collected from the brain or spinal cord. Lymphoid depletion was evaluated as present or absent in lymph nodes and the spleen. Thymus was not available for examination in most cases, thus was not scored. Fibrosis, inflammation, necrosis, lipidosis, hemosiderosis, and biliary hyperplasia were evaluated in the liver. To assess nutritional status, presence or absence of thin epicardial adipose tissue, pancreatic zymogen depletion, and retained gastric superficial epithelial cell layers was determined in UME dolphins. The presence or absence of suspected morbillivirus infections or neurobrucellosis was determined based on available diagnostic tests and the presence or absence of representative histologic lesions in pulmonary, lymphoid, and central nervous system tissues similar to lesions previously described [16, 17, 27].
Cause of death
Causes of death for UME and reference dolphins were acquired from original histopathology reports generated by a veterinary pathologist. Causes of death were based on histologic findings, gross necropsy reports, and ancillary test results. To ease comparisons among groups in the current study, causes of death were grouped into nine general categories: infectious, unknown—poor body condition, unknown—body condition not noted as likely contributor, trauma, organ failure, food obstruction/fish spine injury, maternal separation, and neoplasia. Cause of death was listed as multifactorial if more than one primary cause of death was noted by the pathologist. The prevalence of cause of death categories were determined for all UME dolphins, the Barataria Bay UME dolphin subset, UME dolphins with thin adrenal gland cortices, UME dolphins with primary bacterial pneumonia, all reference dolphins, and the standardly scored reference dolphin subset.
Diagnostic tests
Morbillivirus, marine biotoxin, and Brucella tests were conducted on UME dolphins when appropriate tissues were available. Lung or lung-associated lymph node tissues, frozen and stored at -80°C, were tested for dolphin morbillivirus using a previously validated PCR assay [16]. Liver, feces, or gastric samples were analyzed for brevetoxin using an enzyme-linked immunosorbent assay (ELISA) and/or liquid chromatography—mass spectrometry (LC-MS), and analyzed for the presence of domoic acid by tandem mass spectrometry coupled with LC-MS/MS (MS) [12, 28, 29]. A Brucella PCR assay was used to test for Brucella in multiple tissues including lung, lung-associated lymph node, reproductive tract and/or central nervous system tissues frozen and stored at -80°C. Numbers and types of tissues tested varied by individual and were based upon tissues that were collected and available for testing. Brucella DNA was extracted from tissue samples using a commercially available kit (DNeasy, Qiagen) following the manufacturer’s instructions. Samples were tested for Brucella DNA using a semi-quantitative real-time PCR with standard reagents (Gene Expression Master Mix, Applied Biosystems) and a minor groove binding probe that cross-reacts with known terrestrial and marine Brucella species (University of Illinois, Zoological Pathology Program Molecular Diagnostic Laboratory). Presence of Brucella DNA was confirmed in all equivocal and positive samples by PCR amplification and sequencing of a 135 bp fragment of the Brucella 16SrRNA gene and/or sections of the outer membrane protein (OMP) 2 gene according to published protocols [30]. Nucleotide sequences were determined in both directions on an automated sequencer (Applied Biosystems 3730XL).
As a follow up to UME dolphins in which bacterial pathogens were suspected in the lung, lung samples from five dolphins were tested for the presence of bacterial pathogen DNA using a commercially available kit (MicroSeq 500 16S rDNA Bacterial Identification System, Life Technologies, Grand Island, NY, USA) according to the manufacturer’s instructions and compared nucleotide sequences using the MicroSeq ID Analysis Software. Lung samples from UME dolphins were also tested for the presence of Nocardia DNA using previously published protocols [31]. Necropsy culture swabs and tissues were also collected for classical microbial culture techniques. Isolated bacteria from lesions that were not believed to be due to overgrowth contamination, based upon the pathologists’ interpretation of tissues, were reported.
Data management
A Microsoft Excel datasheet was used to enter the study data in a standardized way into an analyzable format. Variables in the datasheet included the unique animal field identifier, total body length (cm), sex, and state, county, and date of observed dolphin stranding. Diagnostic test results, including samples tested for morbillivirus, Brucella, and biotoxins were included. Histology variables described in the section above were entered into the database using standardized terms.
Statistics
Data were analyzed using World Programming System (WPS 3.1) (World Programming Ltd., United Kingdom). The following four study groups were used for analysis: all UME dolphins, Barataria Bay subset UME dolphins, all reference dolphins, and the standardly scored subset reference dolphins. Descriptive statistics included stranding observation dates and state, sex, and body length. Body lengths and sex distribution were compared between UME and reference dolphins using a Wilcoxon rank-sum test and chi-square test, respectively. Throughout the UME and reference dolphin comparisons, sample sizes varied based upon information available, tissues collected, and tests conducted on individual UME and reference dolphins.
The prevalence of targeted histologic lesions and causes of death, described above, were compared between UME and reference dolphins using chi-square tests and odds ratios or Fisher’s exact tests (if compared categories had less than or equal to five values). To further assess the potential influence of sex and body length on those lesions with significant differences between standardly scored cases and references, a general linear model was used which included sex and body length as covariates and dummy, binary variables for the presence or absence of lesions. Significance was defined as a P value less than 0.05.
Results
UME and reference dolphin demographics
Forty-six fresh dead dolphins that stranded between June 2010 and December 2012 were included in the study (Table 1). A total of 106 reference dolphins were fresh dead and had tissues and/or histologic reports available, including dolphins that stranded along the Gulf coast of Florida (2002–2009), North Carolina (2004), South Carolina (1996–2012) and Texas (2003–2005). There were no significant differences in body length or sex when comparing GoM UME and reference dolphins (Table 2). Using a general linear model, body length and sex were reconfirmed as non-significant covariates for lesions with significant differences between UME and reference dolphins. Accordingly, potential physiologic influences of age and sex did not confound interpretation.
Table 2. Prevalence comparisons of lesions detected using histologic examination for fresh dead, stranded common bottlenose dolphins (Tursiops truncatus) among 1) UME cases from Louisiana, Mississippi, and Alabama, 2) Barataria Bay, Louisiana UME subset, 3) all reference dolphins, and 4) slide-reviewed and standardly scored reference dolphin subset.
doi:10.1371/journal.pone.0126538.t002
Adrenal gland
Among UME dolphins in which appropriate adrenal gland tissue was available for evaluation, one-third (12/36) had a thin adrenal gland cortex and low corticomedullary ratio (less than 0.447), including 9 (50%) Barataria Bay dolphins (Table 2). UME dolphins were more likely to have a thin adrenal gland cortex compared to reference dolphins (33% versus 7%, P = 0.003,Table 2). Adrenal glands with a thin cortex had reduced numbers of cells with only a thin band of cortex remaining (Fig 1). In most cases, distinction between the zona fasciculata and reticularis was difficult. Although cells in the zona fasciculata region appeared reduced in number, it was not possible to distinguish whether the zona glomerulosa and zona reticularus were also affected. In the most severely affected cases, corticocytes were small, though cell size varied greatly within and among the cortex of all individuals. Some dolphins without a thin cortex had small corticocytes. There was no evidence of hemorrhage, necrosis, or fibrosis in affected adrenal glands. The size of the adrenal medulla was within normal limits for all dolphins.
Fig 1. Hematoxalin and eosin stained sections of adrenal glands from common bottlenose dolphins (Tursiops truncatus) C = cortex; M = medulla.
A. Normal adrenal gland from a subadult male dolphin that stranded fresh dead along the west coast of Florida in 2003. Bar = 1 mm. B. Adrenal gland from a subadult male dolphin that stranded in Barataria Bay, Louisiana in 2011. Note the thin adrenal cortex, especially in the fasciculata region. Bar = 1mm.
doi:10.1371/journal.pone.0126538.g001
The proportion of stranded dolphins with thin adrenal gland cortices was similarly high by year (June–December 2010 = 3/9, 33%, 2011 = 4/15, 27%, 2012 = 5/12, 42%) (Fig 2). Of UME dolphins with a thin cortex, 4 (33%) had a concurrent primary bacterial pneumonia. None of the thin adrenal cortex UME dolphins had evidence of morbillivirus infection, and one case hadBrucella meningoencephalitis. None of the UME dolphins with a thin cortex had depleted epicardial adipose tissue, an indicator of advanced, poor nutritional state. The most common noted cause of death among dolphins with a thin adrenal cortex was unknown death not attributed to poor body condition (42%).
Fig 2. Prevalence of key histologic lesions among fresh dead, stranded common bottlenose dolphins (Tursiops truncatus) by year in Louisiana, Mississippi & Alabama: June 2010 to December 2012.
The gray line represents the percentage of lesion prevalence among standardly scored reference dolphins. All years had prevalence of lesions greater than the reference group (P > 0.05).
doi:10.1371/journal.pone.0126538.g002
Among all UME dolphins, mild adrenal corticocyte vacuolation was noted in three (7%) dolphins, and adrenal gland inflammation was noted in two (4%); one of these dolphins had intralesional bacteria associated with disseminated infection. There was no significant difference in the prevalence of corticocyte vacuolation between UME and reference dolphins (7% versus 12%, P = 0.49). No other pathogens were noted in any other UME dolphin adrenal glands.
Lung
Of the UME dolphins, 22% had a primary bacterial pneumonia. In 7 (70%) of UME dolphins with primary bacterial pneumonia, the condition either caused or contributed significantly to death (Tables 3 and 4). The prevalence of primary bacterial pneumonia was higher than reference dolphins (2%) (P = 0.003). Of the 10 UME dolphins with primary bacterial pneumonia, 5 (50%) had severe pneumonia. Overall, UME dolphins had a higher prevalence of severe pneumonia compared to standardly scored references (16% versus 2%, P = 0.03). Primary bacterial pneumonia was most common in UME dolphins from June to December 2010 and was less prevalent during 2011 and 2012 (2010 = 4/10, 40%, 2011 = 3/20, 15%, 2012 = 3/16, 19%) (Fig 2). All UME years examined, however, had a higher prevalence of primary bacterial pneumonia compared to reference dolphins (Fig 2, line indicating prevalence among standardly scored reference dolphins). Six (60%) UME dolphins with primary bacterial pneumonia had bronchopneumonia. UME dolphins overall had a higher prevalence of inflammatory lung infiltrate characterized as granulomatous compared to the standardly scored reference dolphins (78% versus 57%, P = 0.03, Table 3).
Table 3. Prevalence comparisons of lung lesions detected using histologic examination for fresh dead, stranded common bottlenose dolphins (Tursiops truncatus) among UME dolphins from 1) Louisiana, Mississippi, and Alabama, 2) slide-reviewed and standardly scored reference dolphin subset, 3) Barataria Bay, Louisiana UME dolphin subset, 4) and all reference dolphins.
doi:10.1371/journal.pone.0126538.t003
Table 4. Prevalence comparisons of causes of death for fresh dead, stranded common bottlenose dolphins (Tursiops truncatus) among 1) unusual mortality event cases from Louisiana, Mississippi, and Alabama, 2) slide-reviewed and standardly scored reference dolphin subset, 3) Barataria Bay, Louisiana UME dolphin subset, and 4) all reference dolphins.
doi:10.1371/journal.pone.0126538.t004
In UME dolphins with primary bacterial pneumonia, bronchioles and alveolar spaces were often filled with viable and necrotic neutrophils (i.e. suppurative) and fewer macrophages. Bacteria were extracellular or intracellular within neutrophils and macrophages. In some cases, there was extensive necrosis. In the most severe cases, large accumulations of inflammatory cells completely obscured normal pulmonary parenchyma. Inflammatory cells and bacteria were encircled by dense bands of fibrous connective tissue and there were multifocal abscesses (Fig 3). In several cases, inflammatory cells surrounded bacterial colonies that were bordered by radiating, bright eosinophilic club-shaped material (Splendore-Hoeppli material).
Fig 3. Hematoxalin and eosin (HE) stained section of lung from an adult female common bottlenose dolphin (Tursiops truncatus) that stranded in Barataria Bay, Louisiana in December 2011 with primary bacterial pneumonia.
Airspaces and septae are obscured by accumulations of neutrophils and fibrous connective tissue that surround large bacterial colonies (arrows). Bar = 500 μm.
doi:10.1371/journal.pone.0126538.g003
Among UME dolphins with primary bacterial pneumonia, bacteria within lung lesions included coccobacilli (n = 3), cocci (n = 2), and gram positive acid fast negative filamentous bacteria (n = 2). Pulmonary Escherichia coli, Staphylococcus aureus, and Streptococcus spp. Group G infections were confirmed via bacterial culture of lung tissue in UME cases. Staphylococcus aureus and Streptococcus spp. infections were associated with sepsis. Another UME dolphin with primary bacterial pneumonia had concurrent PCR-confirmed Brucella meningoencephalitis, although Brucella PCR on lung tissue was negative.
Among UME dolphins overall, two dolphin lungs were positive for Brucella sp. via PCR. Histologically, these dolphins did not have lung lesions consistent with active brucellosis. Additional bacterial PCR assays used in other primary bacterial pneumonia cases were unsuccessful in amplifying any single pathogenic bacterial species from affected lung tested, likely due to postmortem bacterial overgrowth.
Of UME dolphins with primary bacterial pneumonia and had a measurable adrenal gland, 4n/9 (44%) had a thin adrenal gland cortex. One of 10 (10%) UME dolphins with primary bacterial pneumonia and known cardiac histologic evaluation had depleted cardiac adipose tissue, 3 of 6 (50%) had zymogen depletion, and 2 of 6 (33%) had retained gastric epithelium. Other common pulmonary lesions among UME and reference dolphins included lungworm pneumonia, pulmonary fibrosis, and angiomatosis. The most common cause of pneumonia amongst both UME and reference dolphins was primary lungworm infection (Table 3). Reference dolphins, however, were more likely to have this common cause of pneumonia than the UME dolphins (62% versus 41%, respectively, P = 0.005).
Lymphoid tissues
UME dolphins had a higher prevalence of lymphoid depletion in either or both the spleen or lymph node than reference dolphins (Table 2). When lymphoid depletion was present in either the lymph nodes or the spleen, both B lymphocyte (lymphoid follicles in the spleen and lymph node and medullary cords of the lymph node) and T lymphocyte (periarterior lymphoid sheaths in the spleen and the paracortex of the lymph nodes) regions were affected in the majority of the UME cases. Affected lymphoid follicles had a thin mantle zone often depleted of small lymphocytes. Of the five UME cases with both splenic and lymph node depletion, three died from morbillivirus infections, one died from non-Brucella bacterial meningoencephalitis, and another died from generalized debilitation associated with poor body condition.
Central nervous system
Meningitis or meningoencephalitis were equally common in UME and reference dolphins (20% and 30%, respectively) (Table 2). Central nervous system (CNS) inflammation among UME dolphins was characterized as mild (n = 1), moderate (n = 5), and severe (n = 3). The most common pathogen category associated with meningoencephalitis was bacterial (5, 11% of UME dolphins), which was similar to the reference dolphins (4, 9%) (Table 2). Three (7%) UME dolphins had Brucella-associated meningoencephalitis. Two of the UME dolphins with encephalitis or meningoencephalitis had a thin adrenal gland cortex, and one had a primary bacterial pneumonia.
Liver
The prevalence of hepatic lesions, including fibrosis, necrosis, hepatitis, hemosiderosis, lipid deposition, and hepatobiliary hypertrophy, was similar among UME and reference dolphins (Table 2). The prevalence of moderate to severe hepatic fibrosis (31% versus 36%, P = 0.65) and inflammation (2% versus 7%, P = 0.62) were also comparable between the UME and reference dolphins. Fibrosis and inflammation had a periportal distribution in the majority of the dolphins from both groups, and lesions were most often mild to moderate in severity. Two UME cases were identified with centrilobular hepatocellular vacuolation, degeneration, necrosis, and loss with stromal collapse (Fig 4). Both of these dolphins stranded in Barataria Bay, one in June 2011 and one in July 2012. The June 2011 stranded dolphin was found live and externally oiled. The July 2012 stranded dolphin had evidence of jaundice on gross examination consistent with significant liver disease.
Fig 4. Hematoxylin and eosin (HE) stained section of liver from a juvenile dolphin that stranded fresh dead in Barataria Bay in July 2012.
There is severe centrilobular hepatocellular vacuolation, necrosis, and loss with lobular collapse (arrow). Star indicates a portal triad. Arrow head indicates unaffected liver parenchyma for comparison. Bar = 500μm.
doi:10.1371/journal.pone.0126538.g004
Causes of death
The most common cause of death for UME dolphins was infectious disease (39%), and UME dolphins were four times more likely to die from infectious causes than the reference dolphins (14%, P = 0.01) (Table 4). The most common causes of death for Barataria Bay dolphins were infectious disease (27%) and unknown, not attributed to poor body condition (32%) (Table 4). An unknown cause of death not attributed to poor body condition was most common in UME dolphins with a thin adrenal gland cortex (42%, 5/12). Only three of 19 (16%) UME dolphins that died from infectious causes (and had available adrenal gland for measurement) had a thin adrenal gland cortex; of all 19 UME dolphins that died from infectious causes, eight (42%) had a primary bacterial pneumonia, and five (26%) died from morbillivirus infections.
Nutritional status
Of UME dolphins in which appropriate tissues were available to asses nutritional status, 2/42 (5%) had thin epicardial adipose tissue indicative of emaciation. There were also indicators of inanition by pancreatic zymogen depletion and retained superficial gastric epithelium in 5/28 (18%) and 7/27 (26%) of dolphins, respectively.
Morbillivirus, Brucella, and marine biotoxins
A total of 6/43 (14%) lung and 5/33 (15%) CNS samples from UME dolphins were positive for morbillivirus using PCR, with a total of 8 (19%) UME dolphins with tissues positive for morbillivirus. Five of these eight UME cases had relevant histologic lesions in tissue from which morbillivirus was identified. Five UME morbillivirus PCR positive cases stranded in 2011 and three in 2012 in multiple states. Most (6 of 8) UME dolphins with morbillivirus infections were either calves, juveniles, or subadults. Lesions noted in morbillivirus-affected dolphins included splenic and lymph node lymphoid depletion, syncytial cells, pneumonia, and lymphocytic encephalitis. Two cases had concurrent fungal infections.
Three cases of brucellosis, all with moderate to severe Brucella-associated lymphoplasmacytic meningoencephalitis and confirmed identification of Brucella using PCR or culture, were identified among UME dolphins. The prevalence of brucellosis based upon histopathology and ancillary testing in UME dolphins (6%) was similar to those in the standardly scored subsets (2/42, 5%, P = 0.68). There were also no differences in the prevalence of Brucella detection using PCR on lung or lung-associated lymph node between UME and reference dolphins (5% and 8%, respectively, P = 0.58, Table 3). All UME dolphins with brucellosis were males.
Fecal, gastric content, or liver samples from twenty-eight of the UME dolphins included in this study were tested for brevetoxin and/or domoic acid. Of 26 UME dolphins tested for brevetoxin, one dolphin had detectible levels of brevetoxin by ELISA (2 ng/g) but was negative by LC-MS. Of 27 UME dolphins tested for domoic acid, three were positive at low concentrations (all were 8 ng/g). All positive results were consistent with background exposures [12].
Discussion
To our knowledge, adrenal cortical atrophy as found in this study has not been previously described in free-ranging cetaceans, including bottlenose dolphins previously studied in the northern GoM [32]. The normal corticomedullary ratio of dolphin adrenal glands has been determined to be approximately 1:1 [24]. Thus, the discovered high prevalence of adrenal cortical atrophy in dolphins stranding during the ongoing GoM UME may be part of a syndrome that has not been previously reported in dolphins during mortality events. The prevalence of adrenal cortical atrophy identified in this study is consistent with the high prevalence (approximately 50%) of live Barataria Bay dolphins with evidence of hypoadrenocorticism assessed during 2011, including a relatively high proportion of dolphins with low blood cortisol, aldosterone, and glucose [11]. Follow up evaluation of adrenal glands from stranded dolphins in subsequent years will help to determine the persistence of adrenal insufficiency observed relative to the timing of the UME and the concurrent DWH oil spill.
There are a number of different causes of adrenal insufficiency in mammals, including autoimmune disease, metastatic neoplasia, fungal infections, stress, trauma, miliary tuberculosis, corticosteroid toxicity, and contaminant exposure [33]. Additionally, infection with phocine herpesvirus-1 has been demonstrated to cause adrenal cortical necrosis in marine mammals [34]. In the current study, only 2 of 46 UME dolphins had inflammation in the adrenal gland, and with the exception of one case with a disseminated bacterial infection, neither infectious agents nor neoplasia were identified in UME dolphin adrenal glands. Further, there was no histologic evidence of autoimmune adrenalitis or neoplasia in any UME dolphin adrenal glands, indicating that adrenal cortical atrophy in UME dolphins was not due to direct infection of the adrenal gland, autoimmune disease, or neoplasia.
In humans, chronic demand on the adrenal glands, including chronic illness, has been postulated to lead to cortical thinning and potential adrenal exhaustion associated with lipid depletion of the fasciculata cells [35,36]. Previous evaluations of adrenal glands from stranded GoM dolphins from Texas with both acute and chronic disease have been conducted, but no cases of adrenal cortical atrophy were identified [32]. Instead, adrenal glands of dolphins dying from chronic disease (likely chronically stressed individuals) were significantly heavier, and corticomedullary ratios were significantly higher than those dying from acute disease or acute trauma. Findings from Clark et al. (2006) suggest that adrenal gland enlargement and cortical hyperplasia are common responses to chronic stress and disease in bottlenose dolphins, similar to that noted in other cetaceans and other mammalian species [24, 32, 37–39]. Of the 12 UME dolphins with a thin adrenal gland cortex, one-third had primary bacterial pneumonia, leaving the majority of adrenal cortex cases without evidence of active or chronic infections. Further, none of the UME dolphins with a thin adrenal gland cortex had depleted cardiac adipose tissue, indicating that UME dolphins were not in an advanced, debilitated nutritional state. These results do not support general infection or chronic poor body condition as underlying causes of adrenal gland cortex depletion.
Although the effects of polycyclic aromatic hydrocarbon (PAHs) on the hypothalamus-pituitary-adrenal (HPA) axis are poorly understood, the adrenal gland is reported to be the most common endocrine organ to exhibit lesions with exposure to toxigenic chemicals [40, 41]. In general, mechanisms of direct adrenal toxicity include impaired steroidogenesis, activation of toxins by cytochrome p450 enzymes generating reactive oxygen metabolites, DNA damage, and exogenous steroid action [42]. The adrenal gland can be a significant site for metabolism of PAHs, thus increasing the adrenal gland to exposure from these contaminants and their metabolites [43].
Several studies have shown that PAHs or oil can affect the HPA axis and adrenal gland function. Hypoadrenocorticism has been reported in mink fed either bunker C or artificially weathered fuel oil [44,45]. In these mink, adrenal cortical hypertrophy with vacuolation of corticocytes was detected histologically. These studies, however, did not monitor changes in response to higher level exposure and/or over longer periods of time. Chemicals that induce adrenal cortical vacuolar degeneration can lead to loss of adrenocortical cells due to necrosis and adrenal cortical atrophy. It is possible PAHs may act in a similar fashion [42]. Naphthalene, a common PAH associated with crude oil, reduced plasma corticosterone in mallard ducks following ingestion of petroleum-contaminated food, and a similar acute decrease in cortisol was detected in exposed eels [46, 47]. House sparrows exposed orally to 1% crude oil from the GoM exhibited decreases in cortisol in response to stressors or to adrenocorticotropin hormone injection [48].
Mammalian exposure to PAHs can greatly increase hepatic metabolism of other compounds (e.g. 7,12-dimethylbenz(α)anthracene), which in turn can cause targeted and severe injury to the adrenal gland, including necrosis and hemorrhage [49–51]. Removal of the inciting chemical, if the adrenal cortical injury is not too advanced, may result in regained function and resolved lesions characterized by fibrosis, atrophy, nodular regeneration or calcification [42, 47,51]. Ultrastructural analysis can be beneficial in helping to identify direct toxic damage. Unfortunately, optimally fixed, minimally autolyzed tissue from affected dolphin adrenal glands was not available for ultrastructural analysis. The lack of adrenal lesions beyond cortical atrophy suggests, however, that potential chemical effects may be higher in the HPA axis [52].
During and following the DWH oil spill, significantly elevated PAH levels were detected in the coastal GoM waters, including Louisiana, Mississippi, and Alabama [53]. These locations coincide with the states most impacted by the ongoing UME since the DWH oil spill [1]. Thus, northern GoM dolphins’ exposures to DWH spill-associated PAHs, especially in Louisiana and Mississippi, may account for the observed effects on adrenal function found in both live and dead dolphins [11]. Given the lack of evidence of alternative causes of adrenal cortical atrophy and the high prevalence of this lesion among stranded dolphins following the DWH oil spill, the leading hypothesis is that exposure to contaminants from the DWH oil spill led to chronic injury of the adrenal gland cortex at least through 2012.
Chronic adrenal insufficiency (CAI) is a life-threatening disease that can lead to adrenal crisis and death in mammals [53]. Adrenal crises in people with CAI are triggered by infections, fever, major pain, psychological distress, heat, and pregnancy [54]. Cold temperatures can also increase the risk of death among animals with CAI. Angora goats with a genetically-driven high incidence of primary CAI lack proper cortisol and glucose response and, as such, are susceptible to die-offs from cold stress [54, 55]. GoM dolphins were exposed to colder than normal temperatures during early 2011, and if those dolphins from the UME had pre-existing CAI, they may have been at higher risk of cold stress-related deaths [6]. This hypothesis is further supported in that dolphins have a compensatory adrenal response in cold temperatures, including increased cortisol levels, presumably to help generate metabolic heat [56]. Adrenal crisis may have been the cause of death for many of the UME dolphins with adrenal cortical atrophy following stress events to which a healthy dolphin could have otherwise adapted. In addition to the cold weather during 2011, adrenal crisis could have also been precipitated by late-term pregnancies and infections, including bacterial pneumonia [57].
Compared to reference dolphins, UME dolphins were more likely to have a primary bacterial pneumonia. Many of these pneumonias were much more severe than bacterial pneumonias in the reference dolphins. These findings are consistent with the high prevalence of moderate to severe lung disease detected in live Barataria Bay dolphins [11]. During the DWH oil spill and response period, numerous dolphins, including dolphins in Barataria Bay, were observed swimming through visibly oiled waters and feeding in areas of surface, subsurface, and sediment oiling [11]. As mentioned, the presence of increased coastal PAH levels associated with the DWH oil spill, especially near Grand Isle, Louisiana in Barataria Bay, have been confirmed, indicating an increased risk of inhaled PAHs in dolphins [4]. Given that the dolphin's blowhole is at the surface of the water, chemicals, including volatile PAHs, could have been readily inhaled. In other animals, inhaled PAHs can irritate airways, denude mucosal surfaces, and cause peribronchial inflammation and systemic toxicity [58]. Damaged epithelium and cilia, in turn, can severely impair immune defenses.
In other animals, the severity of chemical inhalation injury is dependent on breathing patterns, in which deep breaths increase injury to tissues deeper within the lung [59]. This pattern is of particular importance given the dolphin's respiratory anatomy and physiology. While humans exchange approximately 10 to 20% of air with each breath, dolphins exchange 75 to 90% of deep lung air [60–63]. They also lack nasal turbinates and cilia to filter the air prior to reaching the lungs, and have deep inhalations followed by a breath hold that provides potential for more prolonged contact and exchange between air-borne particulates and the blood [60–63]. All of these factors would likely amplify the effects of inhaled chemical irritants in dolphins compared to observations and studies involving other mammals.
The severe bacterial pneumonias found in UME dolphins could represent a chronic sequelae to hydrocarbon inhalation or aspiration, or have been secondary to PAH induced immune system compromise. The most common sequela to hydrocarbon inhalation and ingestion in humans and animals are aspiration pneumonia and pneumonia often involving the bronchioles [64–68]. Inhaled hydrocarbon vapors or aspirated hydrocarbons may cause necrosis of bronchial and bronchiolar epithelium, and pneumocyte and alveolar septal necrosis which leads to inflammation and secondary infection [64–66]. During the 2007 firestorm in San Diego, dolphins and people living in San Diego Bay area were exposed to high levels of PAHs [69, 70]. The month following the fires, these dolphins demonstrated decreased absolute and percent neutrophils [70]. This change indicated that dolphins exposed to PAHs through inhalation may have had a compromised immune response and an increased risk of acquiring bacterial pneumonia.
In addition to inhalation risks, hydrocarbon ingestion can lead to gastrointestinal mucosal irritation, vomiting or regurgitation, and resultant aspiration pneumonia. Cattle that ingest petroleum develop bacterial pneumonia due to chemical-induced regurgitation and/or aspiration [67,68]. Correspondingly, based on histologic examination, one dolphin that stranded during June 2010 in Mississippi during the DWH oil spill had suspected aspiration pneumonia, secondary bacterial infection, ulcerative tracheitis, and ulcerative gastritis with edema. Both the tracheal and gastric lesions, although non-specific, could have resulted from mucosal irritation, such as may occur with toxin ingestion.
Though there was no difference in prevalence of liver lesions when comparing UME and reference dolphins in this study, two UME dolphins had similar severe centrilobular liver lesions characterized by hepatocyte loss, necrosis and vacuolation that could potentially be associated with toxin exposure. Both of these dolphins stranded in Barataria Bay. Hepatocellular vacuolation, degeneration and necrosis have been associated with exposure to crude oil and benzo[a]pyrene (BaP) [71 72]. Hepatotoxic liver injury may occur due to xenobiotic metabolism of substances producing injurious metabolites and lesions most often occur in the centrilobular zones where hepatocytes have the highest concentration of cytochrome p450 enzymes [71]. Other rule-outs for centrilobular degeneration and necrosis include hypoxia, severe or precipitous anemia (e.g. hemolytic anemia), chronic heart disease, or circulatory failure associated with septic shock [72, 73]. There was no other evidence of hypoxia, hemolytic anemia or heart disease in either of the affected dolphins and lesions were more chronic than would be expected secondary to acute hypoxia or shock. Some oiled sea otters that died following the Exxon Valdez oil spill had centrilobular hepatic necrosis, though whether the lesions were due to direct toxic insult or secondary to anemia is unclear [74]. Biliary or periportal inflammation and fibrosis secondary to infection by the trematode Campula spp. are common hepatic lesions noted in a number of cetacean species, and periportal lesions noted in both UME and reference dolphins were consistent with the chronic sequelae of biliary trematode infection [75].
This study did not support that previously documented or suspected contributing factors for GoM dolphin UMEs were primary contributors to the ongoing UME among non-perinatal dolphins. All UME dolphins in this case study had biotoxin levels that were below detectable levels except for one with low levels [12]. Relatively few morbillivirus cases were identified among UME cases. In previous known dolphin morbillivirus-associated die-offs, more than 60% of cases tested positive for the virus when using a similar PCR assay [14,16]. Exposure to morbillivirus has been documented in GoM dolphins, and the cases identified in 2011 and 2012 may represent exposure to the virus in a small number of susceptible individuals in the population [76]. Similarly, there were too few brucellosis cases in this study to explain the ongoing UME, with only two cases that had Brucella identified in the lung, demonstrating thatBrucella was not the driver for increased primary bacterial pneumonia. Despite global reports ofBrucella infections in marine mammals, there have been, to date, no documented brucellosis epizootics in cetaceans [17].
Due to the long duration and large scope of the ongoing UME, there may be multiple factors affecting the health of dolphins by region through time. Aside from the DWH oil spill, there were two relatively smaller oil spills that occurred in and around Barataria Bay during this study’s timeframe. Specifically, the T/V Pere Ana C spill in Mud Lake, Louisiana on July 27, 2010 (approximately 7,000 gallons spilled) and the Cedyco Manilla Village Spill in Bayou Dupont, Louisiana which occurred on September 11, 2011 (approximately 10,500 gallons) [77,78]. In comparison, however, the DHW oil spill released approximately 126,000,000 gallons and was visible across 40 km and 366,000 m2 of Barataria Bay’s shoreline in decreasing amounts over time for at least 2 years, demonstrating the higher magnitude of the DWH oil spill’s likely impact compared to other spills [7–10].
The Gulf of Mexico has historically had documented dead zones with episodes of seasonal hypoxia associated with nutrient loading from the Mississippi River watershed [79,80]. The geographic area and clusters of dolphin stranding identified from the current UME, however, were not limited to single seasons or specific dead zone hotspots [2]. Further, dead zones are often associated with fish die-offs and habitat loss; the lack of emaciation as the primary contributor to the deaths of dolphins in this study supports that the primary driver of this UME was not loss of prey [80].
The lack of baseline diagnostic and histologic data on fresh stranded dolphins prior to 2010 in the UME area, as well as during the pre-DWH oil spill period, paired with an assumption that stranded dolphins should have similar lesion prevalence regardless of location, are limitations in this study. The surprisingly high number of assessed lesions that were not significantly different between the UME and reference dolphins, however, increased the confidence that the study groups were indeed comparable. Continual assessment of trends and changing disease states over time are needed, however, to better understand the potential roles of multiple contributing factors to dolphin mortality during the ongoing UME.
In summary, UME dolphins had a high prevalence of thin adrenal gland cortices (especially in Barataria Bay dolphins) and primary bacterial pneumonia. These findings are consistent with endocrinologic and pulmonary-based observations of live bottlenose dolphins from health assessments in Barataria Bay during 2011 [11]. Previously documented or suspected contributing factors for GoM UMEs (marine biotoxins, morbillivirus, and brucellosis) were not supported by this study as contributors to the ongoing UME. Due to the timing and nature of the detected lesions, we hypothesize that contaminants from the DWH oil spill contributed to the high numbers of dolphin deaths within this oil spill’s footprint during the northern GoM UME following the DWH oil spill. Direct causes of death likely included: 1) affected adrenal gland cortices, causing chronic adrenal insufficiency, 2) increased susceptibility to life-threatening adrenal crises, especially when challenged with pregnancy, cold temperatures, and infections, and 3) increased susceptibility to primary bacterial pneumonia, possibly due to inhalation injury, aspiration of oil, or perturbations in immune function.
Supporting InformationTable. Raw demographic, histologic, and diagnostic data from a case-reference study (June 2010–December 2012) investigating the potential cause(s) of increased bottlenose dolphin (Tursiops truncatus) deaths in the northern Gulf of Mexico following theDeepwater Horizon oil spill.
Acknowledgments
This work could not have been conducted without the efforts of the Marine Mammal Stranding Network, including personnel from those agencies working on the current northern GoM UME: Louisiana Department of Wildlife and Fisheries (especially staff from the Fisheries Research Lab in Grande Isle), Audubon Aquarium of the Americas, Institute for Marine Mammal Studies, Dauphin Island Sea Lab, Emerald Coast Wildlife Refuge, and Gulf World Marine Park, as well as, Micah Brodsky, Jeremy Hartley, Michelle Kelley, Courtney Nelson Seely, Bob MacLean, and Sandi Maillian. The authors acknowledge support from the northern GoM UME Investigative Team and the past and present members of the Working Group for Marine Mammal Unusual Mortality Events. In addition, we thank the following individuals who helped coordinate stranding response, manage or validate regional stranding data, or helped by reviewing or contributing to portions of this manuscript: Laura Aichinger Dias, Laura Engleby, Sandra Horton, Blair Mase-Guthrie, Lauren Noble, Gina Rappucci, Lori Schwacke, Elizabeth Stratton, Cynthia Smith, Sabrina Stevens, and Fran Van Dolah. This work was part of the Deepwater HorizonNRDA being conducted cooperatively among NOAA, other Federal and State Trustees, and BP.
Author Contributions
Conceived and designed the experiments: SVW KMC JL KT JS DF EF TR. Performed the experiments: SVW KMC JL MK KT JS SF RC CC WH JP MT CF SS RE DF GL HW DR WM. Analyzed the data: SVW KMC JL KT. Contributed reagents/materials/analysis tools: SVW KMC JL SF RC CC WH JP MT CF SS DF GL HW DR EF. Wrote the paper: SVW KMC JL KT JS SF RC CC WH JP MT CF RE DF DR WM EF TR.
References
- 1.Litz J, Baran M, Bowen-Stevens S, Carmichael R, Colegrove K, Garrison L, et al. Review of historical unusual mortality events (UMEs) in the Gulf of Mexico (1990–2009): providing context for the multi-year northern Gulf of Mexico cetacean UME declared in 2010. Dis Aqua Org. 2014;112: 161–175. doi: 10.3354/dao02807
- 2.Venn-Watson S, Garrison L, Litz J, Fougeres E, Mase B, Rappucci G, et al. Demographic clusters identified within the northern Gulf of Mexico common bottlenose dolphin (Tursiops truncatus) unusual mortality event: January 2010–June 2013. PLOS ONE. 2015;10: e0117248. doi: 10.1371/journal.pone.0117248. pmid:25671657
- 3.McNutt MK, Chu S, Lubcheno J, Hunter T, Dreyfus G, Murawski SA, et al. Applications of science and engineering to quantify and control the Deepwater Horizon oil spill. PNAS. 2012;109:20222–20228. doi: 10.1073/pnas.1214389109. pmid:23213225
- 4.Allan SE, Smith BW, Anderson KA. Impact of the Deepwater Horizon oil spill on bioavailable polycyclic aromatic hydrocarbons in the Gulf of Mexico coastal waters. Environ Sci Technol. 2012;46: 2033–2039. doi: 10.1021/es202942q. pmid:22321043
- 5.Antonia FJ, Mendes RS, Thomaz SM. Identifying and modeling patterns of tetrapod vertebrate mortality rates in the Gulf of Mexico oil spill. Aqua Toxicol. 2011;105: 177–179. doi: 10.1016/j.aquatox.2011.05.022
- 6.Carmichael RH, Graham WM, Allen A, Worthy G, Howden S. Were multiple stressors a 'perfect storm' for northern Gulf of Mexico bottlenose dolphins (Tursiops truncatus) in 2011? PLOS ONE. 2012;7: e41155. doi: 10.1371/journal.pone.0041155. pmid:22815950
- 7.Michel J, Owens EH, Zengel S, Graham A, Nixon Z, Allard T, et al. Extent and degree of shoreline oiling: Deepwater Horizon oil spill, Gulf of Mexico, USA. PLOS ONE. 2013; doi: 10.1371/journal.pone.0065087.
- 8.Lin Q, Mendelssohn IA. Impacts and recovery of the Deepwater Horizon oil spill on vegetation structure and function of coastal salt marshes in the Northern Gulf of Mexico. Environ Sci Technol. 2012;46: 3737–3743. doi: 10.1021/es203552p. pmid:22369124
- 9.Silliman BR, van de Koppel J, McCoy MW, Diller J, Kasozi GN, Earl K, et al. Degradation and resilience in Louisiana salt marshes after the BP—Deepwater Horizon oil spill. PNAS. 2012;109: 1123–11239. doi: 10.1073/pnas.1204922109
- 10.Kokaly RF, Couvillion BR, Holloway JM, Roberts DA, Ustin SL et al. Spectroscopic remote sensing of the distribution and persistence of oil from the Deepwater Horizon spill in Barataria Bay marhes. Remote Sens Environ. 2013;129: 210–230. doi: 10.1016/j.rse.2012.10.028
- 11.Schwacke LH, Smith CR, Townsend FI, Wells RS, Hart LB, Balmer BC, et al. Health of common bottlenose dolphins (Tursiops truncatus) in Barataria Bay, Louisiana, following the Deepwater Horizon oil spill. Environ Sci Tech. 2014;48: 93–103. doi: 10.1021/es403610f. pmid:24350796
- 12.Fire SE, Wang Z, Byrd M, Whitehead HR, Paternoster J, Morton SL. Co-occurrence of multiple classes of harmful algal toxins in bottlenose dolphins (Tursiops truncatus) stranding during an unusual mortality event in Texas, USA. Harmful Algae. 2011;10: 330–336. doi: 10.1016/j.hal.2010.12.001
- 13.Krafft A, Lichy JH, Lipscomb TP, Klaunberg BA, Kennedy S, Taubenberger JK. Postmortem diagnosis of morbillivirus infection in bottlenose dolphins (Tursiops truncatus) in the Atlantic and Gulf of Mexico epizootics by polymerase chain reaction-based assay. J Wildl Dis. 1995;31: 410–415. pmid:8592367 doi: 10.7589/0090-3558-31.3.410
- 14.Lipscomb TP, Kennedy S, Moffett D, Krafft A, Klaunberg BA, Lichy JH, et al. Morbillivirus epizootic in bottlenose dolphins of the Gulf of Mexico. J Vet Diagn Invest. 1996;8: 283–290. pmid:8844569 doi: 10.1177/104063879600800302
- 15.Twiner M J, Flewelling LJ, Fire SE, Bowen-Stevens SR, Gaydos JK, Johnson CK, et al. Comparative analysis of three brevetoxin-associated bottlenose dolphin (Tursiops truncatus) mortality events in the Florida panhandle region (USA). PLOS ONE. 2012;7: 1–19. doi: 10.1371/journal.pone.0042974
- 16.Schulman FY, Lipscomb TP, Moffett D, Krafft AE, Lichy JH, Tsai MM, et al. Histologic, immunohistochemical and polymerase chain reaction studies of bottlenose dolphins from the 1987–1988 United States Atlantic coast epizootic. Vet Pathol. 1997;34: 288–295. pmid:9240837 doi: 10.1177/030098589703400404
- 17.Hernandez-Mora G, Palacios-Alfaro JD, Gonzalez-Barrientos R. Wildlife reservoirs of brucellosis: Brucella in aquatic environments. Rev Sci Tech Off Int Epi. 2013;32: 89–103. pmid:23837368
- 18.Cassle S, Johnson S, Lutmerding B, Jensen E. Pulmonary brucellosis in an Atlantic bottlenose dolphin (Tursiops truncatus) (2009) In Proceedings of the 40th Conference of the International Association of Aquatic Animal Medicine: 2–6 May 2009. San Antonio. 1999:129.
- 19.Dagleish MP, Barley J, Howie FE, Reid RJ, Herman J, Foster G. Isolation of Brucellaspecies from a diseased atlanto-occipital joint of an Atlantic white-sided dolphin (Lagenorhynchus acutus). Vet Rec. 2007;160: 876–6. pmid:17586794 doi: 10.1136/vr.160.25.876
- 20.Davison NJ, Cranwell MP, Perrett LL, Dawson CE, Deaville R, Stubberfield EJ, et al. Meningoencephalitis associated with Brucella species in a live-stranded striped dolphin (Stenella coeruleoalba) in south-west England. Vet Rec. 2009;165: 86–9. pmid:19617615 doi: 10.1136/vetrec.165.3.86
- 21.Miller WG, Adams LG, Ficht TA, Cheville NF, Payeur JP, Harley DR, et al. Brucella-induced abortions and infection in bottlenose dolphins (Tursiops truncatus). J Zoo Wildl Med 1999;30: 100–110. pmid:10367651
- 22.Hoffman RJ. History, goals and achievements of the regional marine mammal stranding network. pp 7–17. In: Reynolds J.E. and Odell D.K. (eds.) Marine Mammal Strandings in the United States. NOAA Technical Report NMFS 98. 1991;157 pp.
- 23.Terio KA, Marker L, Munson L. Evidence for chronic stress in captive but not free-ranging cheetahs (Acinonyx jubatus) based on adrenal morphology and function. J Wild Dis. 2004;40: 259–266. pmid:15362825 doi: 10.7589/0090-3558-40.2.259
- 24.Clark LS, Pfeiffer DC, Cowan DF. Morphology and histology of the Atlantic bottlenose dolphin adrenal gland with emphasis on the medulla. Anat Histol Embryol. 2005;34: 132–140. pmid:15771677 doi: 10.1111/j.1439-0264.2004.00600.x
- 25.Fauquier DA, Kinsel MJ, Dailey MD, Sutton GE, Stolen MK, Wells RS, et al. Prevalence and pathology of lungworm infection in bottlenose dolphins Tursiops truncatus from southwest Florida. Dis Aqua Org 2009;88: 85–90. doi: 10.3354/dao02095
- 26.Turnbull BS, Cowan DF. Angiomatosis, a newly recognized disease in Atlantic bottlenose dolphins (Tursiops truncatus) in the Gulf of Mexico. Vet Path. 1999; 36: 1–28. doi: 10.1354/vp.36-1-28
- 27.Sierra E, Sanchez S, Saliki JT, Blas-Machado U, Arbelo M, Zucca D, Fernandez A. Etiologic agents associated with non-suppurative meningoencephalitis in stranded cetaceans: a retrospective study in the Canary Islands. J Clin Microbiol. 2014; doi: 10.1128/JCM.02906-13.
- 28.Fire SE, Flewelling LJ, Wang Z, Naar J, Henry MS, Pierce MS, et al. Florida red tide and brevetoxins: association and exposure in live resident bottlenose dolphins (Tursiops truncatus) in the eastern Gulf of Mexico, U.S.A. Mar Mamm Sci; 2008;24: 831–844. doi: 10.1111/j.1748-7692.2008.00221.x
- 29.Maucher JM, Briggs L, Podmore C, Ramsdell JS. Optimization of blood collection card method/enzyme-linked immunoassay for monitoring exposure of bottlenose dolphin to brevetoxin-producing red tides. Environ Sci Technol. 2007;41: 563–567. pmid:17310722 doi: 10.1021/es0612605
- 30.Ficht TA, Husseinen HS, Derr J, Bearden SW. Species-specific sequences at theomp2 locus of Brucella type strains. Int J Syst Bact 1996;46: 329–31. pmid:8573514 doi: 10.1099/00207713-46-1-329
- 31.St. Leger JA, Begeman L, Fleetwood M, Frasca S Jr, Garner MM, Lair S, et al. Comparative pathology of nocardiosis in marine mammals. Vet Pathol. 2009;46: 299–308. doi: 10.1354/vp.46-2-299. pmid:19261643
- 32.Clark LS, Cowan DF, Pfeiffer DC. Morphological changes in the Atlantic bottlenose dolphin (Tursiops truncatus) adrenal gland associated with chronic stress. J Comp Pathol. 2006;135: 208–216. pmid:17067620 doi: 10.1016/j.jcpa.2006.07.005
- 33.Oelkers W. Adrenal insufficiency. N Engl J Med. 1996;335: 1206–1212. pmid:8815944 doi: 10.1056/nejm199610173351607
- 34.Gulland FMD, Haulena M, Lowenstine LJ, Munro C, Graham PA, Bauman J, et al. Adrenal function in wild and rehabilitated Pacific harbor seals (Phoca vitulina richardii) and in seals with phocine herpesvirus-associated adrenal necrosis. Mar Mamm Sci. 2006;15:810–827. doi: 10.1111/j.1748-7692.1999.tb00844.x
- 35.Zwemer RL. A study of adrenal cortex morphology. Am J Path. 1936;12: 107–111. pmid:19970248
- 36.Sasano H, Nakamura Y, Moriya T, Suzuki T. Adrenal Cortex. In Endocrine Pathology: Differential Diagnosis and Molecular Advances, Lloyd R.V. (ed). Springer Science + Business Media, LLC 2010. pp 261–262.
- 37.Kuiken T, Hofle U, Bennett PM, Allchin CR, Kirkwood JK, Baker JR, et al. Adrenal cortical hyperplasia, disease, and chlorinated hydrocarbons in the harbor porpoise (Phocoena phocoena). Mar Poll Bull. 1993;26: 440–446. doi: 10.1016/0025-326x(93)90532-o
- 38.Lair S, Beland P, De Guise S, Martineau D. Adrenal hyperplastic and degenerative changes in beluga whales. J Wildl Dis. 1997. 33: 430–437. pmid:9249687 doi: 10.7589/0090-3558-33.3.430
- 39.Rideout BA, Gause GE, Benirschke K, Lasley BL. Stress-induced change and their relationship to reproductive failure in captive nine-banded armadillos (Dasypus novemcinctus). Zoo Bio. 1985;4: 129–137. doi: 10.1002/zoo.1430040206
- 40.Ribelin WE. The effects of drugs and chemicals upon the structure of the adrenal gland. Fund Appl Toxicol. 1984;4: 105–119. doi: 10.1093/toxsci/4.1.105
- 41.Nichols JW. Breen M, Denver RJ, Distefano JJ, Edwards JS, Hoke RA, et al. Predicting chemical impacts on vertebrate endocrine systems. Environ Toxicol Chem. 2011;30:39–51. doi: 10.1002/etc.376. pmid:20963851
- 42.Rosol T. Adrenal gland: structure, function, and mechanisms of toxicity. Toxicol Path. 2001;29: 41–48. pmid:11215683 doi: 10.1080/019262301301418847
- 43.Greiner JW, Kramer RE, Robinson DA, Canady WJ, Colby HD. Interaction of aromatic hydrocarbons and drugs with adrenal microsomal cytochrome P-450 and the guinea pig. Biochem Pharm. 1976;25: 951–955. pmid:1267839 doi: 10.1016/0006-2952(76)90321-x
- 44.Mohr FC, Lasley B, Bursian S. Chronic oral exposure to bunker C fuel oil causes adrenal insufficiency in ranch mink (Mustela vison). Arch Environ Contam Toxicol; 2008;54: 337–347. pmid:17763884 doi: 10.1007/s00244-007-9021-5
- 45.Mohr FC, Lasley B, Bursian S. Fuel oil-induced adrenal hypertrophy in ranch mink (Mustela vison): effects of sex, fuel oil weathering, and response to adrenocorticotrophic hormone. J Wildl Dis. 2010;46: 103–110. pmid:20090023 doi: 10.7589/0090-3558-46.1.103
- 46.Gorsline J, Holmes WN. Adrenocortical function and hepatic naphthalene metabolism in mallard ducks (Anas platyrhynchos) consuming petroleum distallates. Environ Res. 1982;28: 139–146. pmid:7106069 doi: 10.1016/0013-9351(82)90162-1
- 47.Teles M, Pacheco M, Santos MA. Anguilla Anguilla L. liver ethoxyresorufin-deethlylation, glutathione S-tranferase, erthrocytic nuclear abnormalities, endocrine responses to naphthalene and BI-naphthoflavone. Ecotox Environ Safety 2003;55: 98–107. pmid:12706398 doi: 10.1016/s0147-6513(02)00134-3
- 48.Lattin CR, Ngai HM, Romero LM. Evaluating the stress response as a bioindicator of sublethal effects of crude oil exposure in wild house sparrows (Passer domesticus) PLOS ONE. 2014;9: e102106. doi: 10.1371/journal.pone.0102106. pmid:25029334
- 49.Bond B, Orr JW. The effects of a single dose of 7,12-Dimethylbenz(α)anthracene on the epidermis and hair follicles of mice, with notes on concurrent changes in the ovaries and adrenals. Br J Cancer. 1969;23: 188–196. pmid:5768435
- 50.Huggins C, Morii S. Selective adrenal necrosis and apoplexy induced by 7,12—Dimethylben(α)antracene. J Exptl Med. 1961;114: 741–760. doi: 10.1084/jem.114.5.741
- 51.Levin W, Conney AH. Stimulatory effect of polycyclic hydrocarbons and aromatic azo derivatives on the metabolism of 7,12-Dimethylbenz(α)anthracene. Cancer Res. 1967; 27:1931. pmid:4169331 doi: 10.1016/0024-3205(66)90163-9
- 52.Bornstein SR, Chrousos GP. Clinical review 104: adrenocorticotropin (ACTH)- and non-ACTH-mediated regulation of the adrenal cortex: neural and immune inputs. J Clin Endocrinol Metab. 1999;84: 1729–1736. pmid:10323408 doi: 10.1210/jc.84.5.1729
- 53.Arlt W, Allolio B. Adrenal insufficiency. Lancet. 2003;361: 1881–1893. pmid:12788587 doi: 10.1016/s0140-6736(03)13492-7
- 54.Hahner S, Loeffler M, Bleicken B, Drechsler C, Milovanovic D, Fassnacht , et al. Epidemiology of adrenal crisis in chronic adrenal insufficiency: the need for new prevention strategies. Eur J Endocrinol. 2009;162: 597–602. doi: 10.1530/EJE-09-0884. pmid:19955259
- 55.Engelbrecht Y, Herselman T, Louw A, Swart P. Investigation of the primary cause of hypoadrenocorticism in South African angora goats (Capra aegagrus): a comparison with Boer goats (Capra hircus) and Merino sheep (Ovis aries). J Anim Sci. 2000;78: 371–379. pmid:10709928
- 56.Houser DS, Yeates LC, Crocker DE. Cold stress induces an adrenocortical response in bottlenose dolphins (Tursiops truncatus). J Zoo Wildlf Med. 2011;42: 565–571. pmid:22204049 doi: 10.1638/2010-0121.1
- 57.Hills G, Venning EH, Dohan FC, Webster GD, Richardson EM. Pregnancy and adrenocortical function: endocrine studies of pregnancy occurring in two adrenal-deficient women. J Clin Invest. 1954;33: 1466–1480. pmid:13211801 doi: 10.1172/jci103025
- 58.Demling RH. Smoke inhalation lung injury: an update. Eplasty. 2008;8: e27. pmid:18552974
- 59.Lalonde C, Demling R, Brian J, Blanchard J. Smoke inhalation injury in sheep is caused by the particle phase not the gas phase. J Appl Physiol. 1994;77:15–22. pmid:7961227
- 60.Green RF. Observations of the anatomy of some cetaceans and pinnipeds. In: Ridgway SH, editor. Mammals of the sea: biology and medicine. Springfield, IL: Charles C. Thomas Publishers. 1972. p.247–297.
- 61.Irving L, Scholander PF, Grinnell SW. The respiration of the porpoise, Tursiops truncatus. J Cell Comp Physiol. 1941;17: 145–168. doi: 10.1002/jcp.1030170203
- 62.Ridgway SH, Scronce BL, Kanwisher J. Respiration and deep diving in the bottlenose porpoise. Science. 1969;166: 1651–1654. pmid:5360592 doi: 10.1126/science.166.3913.1651
- 63.Ridgway SH, Ed. Mammals of the Sea: Respiration System. Charles C. Thomas, Springfield, Illinois. 1972. pp 260–264.
- 64.Azeez OM, Akhigbe RE, Anigbogu CN. Exposure to petroleum hydrocarbon: implications in lung lipid peroxidation and antioxidant defense system in rat. Toxicol Int. 2012;19: 306–309. doi: 10.4103/0971-6580.103678. pmid:23293471
- 65.Coppock RW, Mostrom MS, Stair EL, Semalulu SS. Toxicopathology of oilfield poisoning in cattle: A review. Vet Human Toxicol. 1996;38: 36–42. pmid:8825749
- 66.Eade NR, Taussig LM, Marks MI. Hydrocarbon pneumonitis. Pediatrics. 1974;54: 351–356. pmid:4606628
- 67.Rowe LD, Dooahite JW, Camp BJ. Toxicity of two crude oils and kerosene to cattle. JAVMA. 1951;162: 61–66.
- 68.Edwards WC. Toxicology of oil field wastes. Hazards to livestock associated with the petroleum industry. Food Animal Prac. 1989;5: 363–374. pmid:2667711
- 69.Bytnerowicz A, Cayan D, Riggan P, Schilling S, Dawson P, Tyree M, et al. Analysis of the effects of combustion emissions and Santa Ana winds on ambient ozone during the October 2007 southern California wildfires. Atmosph Environ. 2010;44: 678–687. doi: 10.1016/j.atmosenv.2009.11.014
- 70.Venn-Watson S, Smith CR, Jensen ED, Rowles T. Assessing the potential health impacts of the 2003 and 2007 firestorms on bottlenose dolphins (Tursiops truncatus) in San Diego Bay. Inhal Toxicol. 2013;25: 481–491. doi: 10.3109/08958378.2013.804611. pmid:23905966
- 71.Agamy E. Histopathological changes in the livers of rabbit fish (Siganus canaliculatus) following exposure to crude oil and dispersed oil. Toxicol Pathol. 2012;40: 1128–1140. doi: 10.1177/0192623312448936. pmid:22659245
- 72.Pancheco M, Santos MA. Biotransformation, genotoxic, and histopathologic effects of environmental contaminants in European eel (Anguilla Anguilla L.). Ecotoxicol and Environ Safety. 2002;53:331–347. pmid:12485576 doi: 10.1016/s0147-6513(02)00017-9
- 73.Cullen JM. Liver. Biliary system, and exocrine pancreas, In Pathologic Basis of Veterinary Diseases, 4th ed, editors McGavin MD and Zachary JF. Mosby Elsevier, St. Louis Missouri. 2007. pp 393–462.
- 74.Lipscomb TP, Harris RK, Moeller RB, Pletcher JM, Haebler RJ, Ballachey BE. Histopathologic lesions in sea otters exposed to crude oil. Vet Pathol. 1993;30: 1–11. pmid:8442322 doi: 10.1177/030098589303000101
- 75.Jaber JR, Arbelo M, Andrada M, Hidalgo M, Gomez-Villamandos JC, Van Den Ingh T, et al. Hepatic lesions in cetaceans in cetaceans stranded in the canary islands. Vet Pathol. 2004;41: 147–153. pmid:15017028 doi: 10.1354/vp.41-2-147
- 76.Duignan P, House C, Odell DK, Wells RS, Hansen LJ, Walsh MT, et al. Morbillivirus infection in bottlenose dolphins: Evidence for recurrent epizootics in the western Atlantic and Gulf of Mexico 1996. Marine Mamm Sci. 1996;12: 499–515. doi: 10.1111/j.1748-7692.1996.tb00063.x
- 77.National Oceanic and Atmospheric Administration. T/V Pere Ana C. Available:http://incidentnews.noaa.gov/incident/8241. Accessed 2010 Jul 27.
- 78.National Oceanic and Atmospheric Administration. Cedyco Manila Village Spill. Available: http://incidentnews.noaa.gov/incident/8358. Accessed 2011 Sep 11.
- 79.Turner RE, Rabalais NN, Justic D. Gulf of Mexico hypoxia: Alternate states and a legacy. Environ Sci Technol. 2008;42: 2323–2327. pmid:18504960 doi: 10.1021/es071617k
- 80.Diaz RJ, Rosenberg R. Spreading dead zones and consequences for marine ecosystems. Science. 2008;321: 926–929. doi: 10.1126/science.1156401. pmid:18703733